

In an effort to overcome these limitations, we previously investigated the hydrodynamic effect of shark skin denticles on flexible pieces of real shark skin that were moved by a robotic flapping mechanical device with amplitudes, frequencies and curvatures that closely approximate those of live sharks ( Oeffner and Lauder, 2012). As the skin of live sharks bends and flexes during swimming and encounters complex time-dependent flows ( Oeffner and Lauder, 2012), analysis of denticle models mounted on rigid plates may have relatively little relevance to the hydrodynamic environment experienced by denticles on freely swimming sharks. Most previous experimental hydrodynamic studies have used simplified denticle models and studied the effects of surface roughness on flat plates, which were held in a rigid, stationary position while in flow. Bioengineering studies of shark skin denticle function have focused on the effects of denticle-like structures using scaled-up models of denticles to study how surface roughness affects drag forces ( Dean and Bhushan, 2010 Lang et al., 2008 Lang et al., 2011 Luchini et al., 1991 Walsh, 1980 Walsh, 1983).

The structure of shark skin denticles and their possible effect on the pattern of water flow over the body has attracted considerable interest from biologists interested in the microstructure and distribution of denticles ( Kemp, 1999 Meyer and Seegers, 2012 Motta et al., 2012 Reif, 1978 Reif, 1982a Reif, 1985), engineers focused on how the surface roughness may reduce drag forces during locomotion ( Bechert et al., 1997 Bechert et al., 2000 Lang et al., 2011), and researchers interested in generating biomimetic models of shark skin to reduce locomotor drag on humans and human-designed structures ( Büttner and Schulz, 2011 Mollendorf et al., 2004).
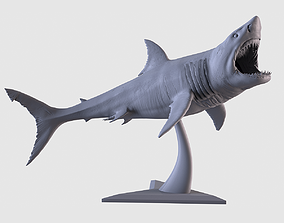
The ability to fabricate synthetic biomimetic shark skin opens up a wide array of possible manipulations of surface roughness parameters, and the ability to examine the hydrodynamic consequences of diverse skin denticle shapes present in different shark species. In addition, a leading-edge vortex with greater vorticity than the smooth control was generated by the 3D printed shark skin, which may explain the increased swimming speeds. For example, at a heave frequency of 1.5 Hz and an amplitude of ☑ cm, swimming speed increased by 6.6% and the energy cost-of-transport was reduced by 5.9%. Compared with a smooth control model without denticles, the 3D printed shark skin showed increased swimming speed with reduced energy consumption under certain motion programs. This flexible 3D printed shark skin model was then tested in water using a robotic flapping device that allowed us to either hold the models in a stationary position or move them dynamically at their self-propelled swimming speed. Using 3D printing, thousands of rigid synthetic shark denticles were placed on flexible membranes in a controlled, linear-arrayed pattern. A three-dimensional (3D) model of shark skin denticles was constructed using micro-CT imaging of the skin of the shortfin mako ( Isurus oxyrinchus).

We present the first study of the design, fabrication and hydrodynamic testing of a synthetic, flexible, shark skin membrane. Although the functional properties of shark skin have been of considerable interest to both biologists and engineers because of the complex hydrodynamic effects of surface roughness, no study to date has successfully fabricated a flexible biomimetic shark skin that allows detailed study of hydrodynamic function.
